Now that we've looked at the basics of laser weapons at sea, it's time for a more detailed analysis of the capabilities of current and projected systems against a variety of targets. This is going to be considerably more math-based and technical than is normal for Naval Gazing, but I spent a bunch of time thinking about laser weapons when I was doing space warfare, and it seemed worth attempting to get a better quantitative handle on what was going on here.
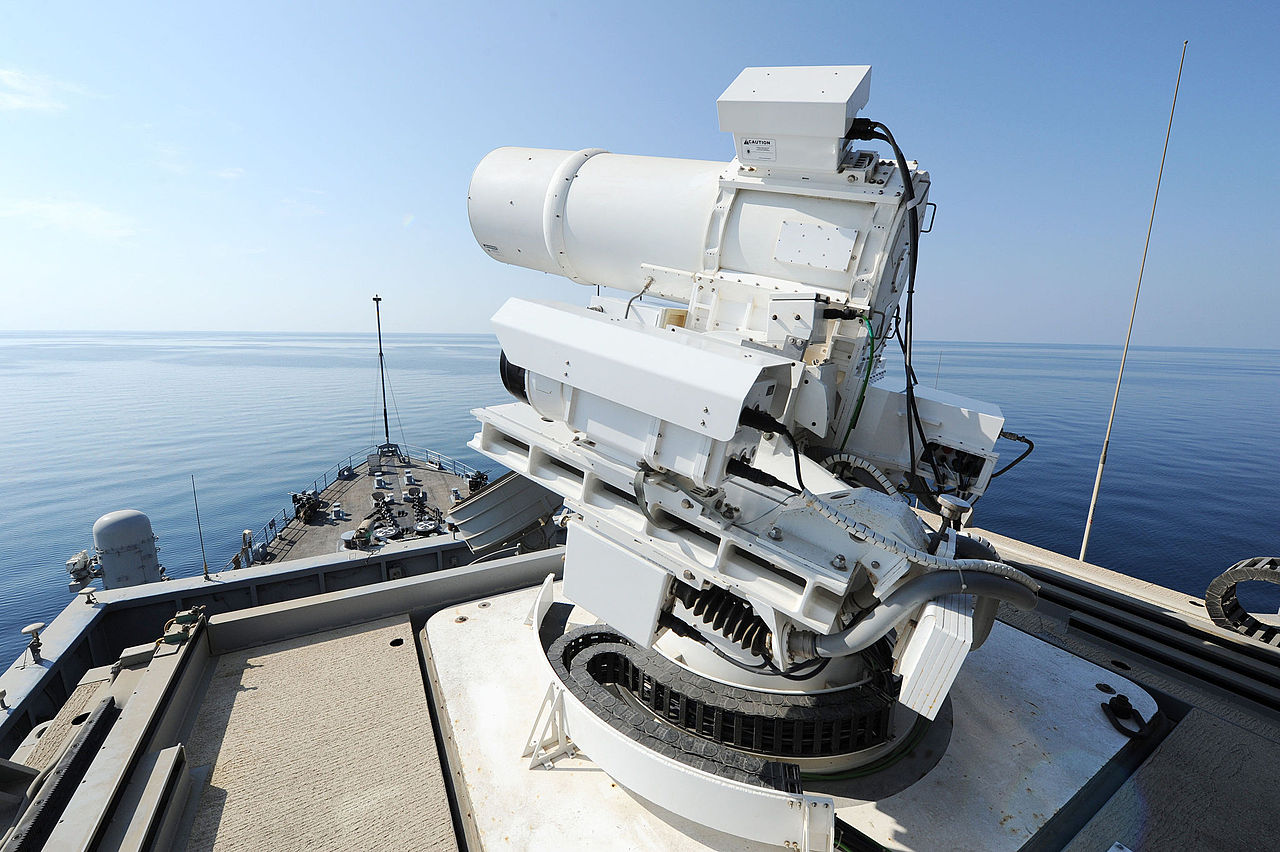
The AN/SEQ-3 aboard Ponce
We'll start with the AN/SEQ-3 Laser Weapon System, which was first deployed aboard USS Ponce, an amphibious ship-turned-afloat base in the Persian Gulf, in 2014. The SEQ-3 is built around six commercial fiber lasers, operating in the IR spectrum and providing a total of 33 kW of power.1 Based on a photograph,2 the main mirror looks to be about 16"/40 cm. Using this paper on laser propagation, I was able to build a spreadsheet that looks to give a good approximation of the performance of various lasers, including the various mechanisms that hinder propagation as discussed last time.3
At this point, we must turn our attention to damage simulation on the target. While there are many ways lasers can damage a target, the only one of interest for our purposes is melting, and using information from Effects of Directed Energy Weapons and How to Build a Laser Death Ray, I added analysis of melting for different materials to the spreadsheet, both if the material is likely to melt and how quickly the material will melt if it does.4 As might be expected, the results will depend heavily on how much of the laser the target will absorb. Bare metals tend to have low absorptivity (~0.1) when solid, but it climbs rapidly when they melt to as high as 0.6-0.8. Other materials tend to be quite high (>0.5).5 Given how few targets encountered at sea are bare metal, I'm going to assume a constant absorptivity of 0.5 for all analysis.6 This suggests that the SEQ-3 will be able to start melting aluminum at around 3.3 km, and would melt around 4.5 mm/sec at that range. In theory, plastic (represented by HDPE) should start melting much further out, as much as 15 km, and with a melt rate of about 0.22 mm/sec.7 Steel is the toughest of the three, with a melting range of around 2.6 km and a melt rate of 2.9 mm/sec.
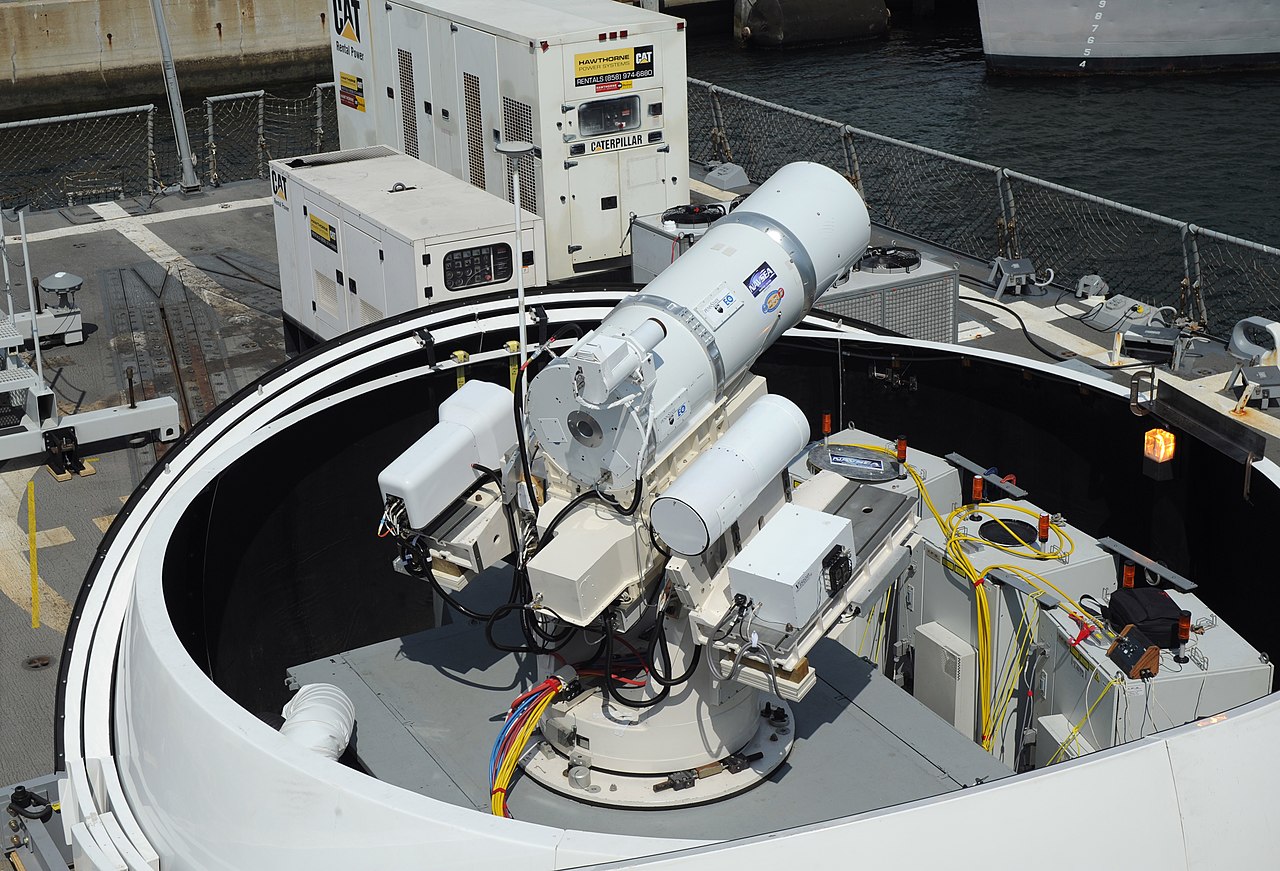
An SEQ-3 temporarily installed on the flight deck of destroyer Dewey
All of this tends to substantiate the Navy's claims with regards to SEQ-3's capabilities, which credit it with being effective against drones and small boats, but not much more. Drones tend to be mostly plastic, a material that SEQ-3 should be reasonably effective against at ranges of 10 km or more. At that point, practical melt rate is probably north of .5 mm/sec, and particularly with the large spot sizes produced at said range, structural failure won't take long. Boats are more likely to be metal, but when the threat is a suicide bomber or an RPG, a few mm/sec is plenty so long as the number of threats is manageable, particularly when unambiguous hostility can be met with more conventional weapons. But what about the next generation? The leader here is the Lockheed HELIOS, which is currently being integrated aboard destroyer Preble, the first laser permanently fitted and integrated aboard a proper warship. Power level is reported to be "above 60 kW", and while no pictures are available, eyeballing from a rendering on Lockheed's website suggests that the mirror will be around 60 cm. A similar analysis using those parameters and a 60 kW laser gives outer ranges of 5.1 km for aluminum, 18 km for HDPE and 4.2 km for steel.8 But work is ongoing to boost HELIOS's power, with the target value of the system being something like 150 kW, and reports indicating that it should be at 120 kW by the time it actually goes to sea. At 150 kW (with the same mirror diameter and other parameters held constant), melt ranges come to 6.7 km for aluminum, 21.3 km for HDPE and 5.7 km for steel. At this point, we're starting to get more into the sorts of systems which could in theory have some anti-missile use, although even a 150 kW HELIOS is still going to be rather limited in that field. A look at melt rates at various ranges is probably instructive:
Range | Aluminum | HDPE | Steel |
---|---|---|---|
10 km | N/A | 1.13 mm/s | N/A |
7.5 km | N/A | 3.08 mm/s | N/A |
5 km | N/A | 10.68 mm/s | N/A |
4 km | N/A | 19.87 mm/s | N/A |
3 km | 5.90 mm/s | 42.09 mm/s | N/A |
2 km | 15.79 mm/s | 112.76 mm/s | 5.65 mm/s |
1 km | 75.00 mm/s | 535.54 mm/s | 26.83 mm/s |
Range | Aluminum | HDPE | Steel |
---|---|---|---|
10 km | N/A | 3.08 mm/s | N/A |
7.5 km | N/A | 8.96 mm/s | N/A |
5 km | 4.76 mm/s | 34.02 mm/s | N/A |
4 km | 9.26 mm/s | 66.12 mm/s | 3.31 mm/s |
3 km | 20.58 mm/s | 146.97 mm/s | 7.36 mm/s |
2 km | 58.13 mm/s | 415.05 mm/s | 20.79 mm/s |
1 km | 291.92 mm/s | 2084.39 mm/s | 104.41 mm/s |
Range | Aluminum | HDPE | Steel |
---|---|---|---|
10 km | N/A | 7.63 mm/s | N/A |
7.5 km | N/A | 22.28 mm/s | N/A |
5 km | 11.87 mm/s | 84.7 mm/s | 4.25 mm/s |
4 km | 23.10 mm/s | 164.95 mm/s | 8.26 mm/s |
3 km | 51.39 mm/s | 366.92 mm/s | 18.38 mm/s |
2 km | 145.22 mm/s | 1036.93 mm/s | 51.94 mm/s |
1 km | 729.67 mm/s | 5210.00 mm/s | 260.97 mm/s |
This clearly shows the vastly increasing power of the laser as it gets closer, although it is worth pointing out some of the limitations of this analysis. As mentioned previously, the math assumes that all melted material is cleared instantly and doesn't carry away any extra heat. This is optimistic even for low melt rates, and becomes even less realistic as melt rates climb, although other effects take over at that point. In theory, the much more sophisticated damage calculator at How to Build a Laser Death Ray suggests that my 1 km 60 kW case would drill through aluminum at 23 cm/s, not too far from the 29 cm/s of my estimation, although it gives only 34 cm/s for HDPE, a vast reduction over my estimate of 208 cm/s. That said, it's also worth pointing out that this analysis assumes the smallest spot size possible, which is unlikely at close range for a variety of reasons. But even with all that said, it seems reasonable to assume that a HELIOS-level laser will have a drill rate of at least 10 cm/sec inside of a kilometer or two, unless the target is specially designed to resist it.
But what does all of this mean against targets in the real world? We've previously looked at things like drones and small boats, which are within the capabilities of SEQ-3 and HELIOS. But these are both quite easy. Drones are unlikely to be a direct threat, meaning that a laser has plenty of time to deal with them, while boats move relatively slowly. While the fastest might top out at more than 70 kts (35 m/s), charging a warship at this speed is best countered with a 25 mm chain gun. A more typical threat is likely to be a converted fishing boat doing maybe 10 m/s. Even if it is heading straight for the ship, the laser should be able to begin doing damage at least 2 km out, two minutes before it gets close enough to be a threat.
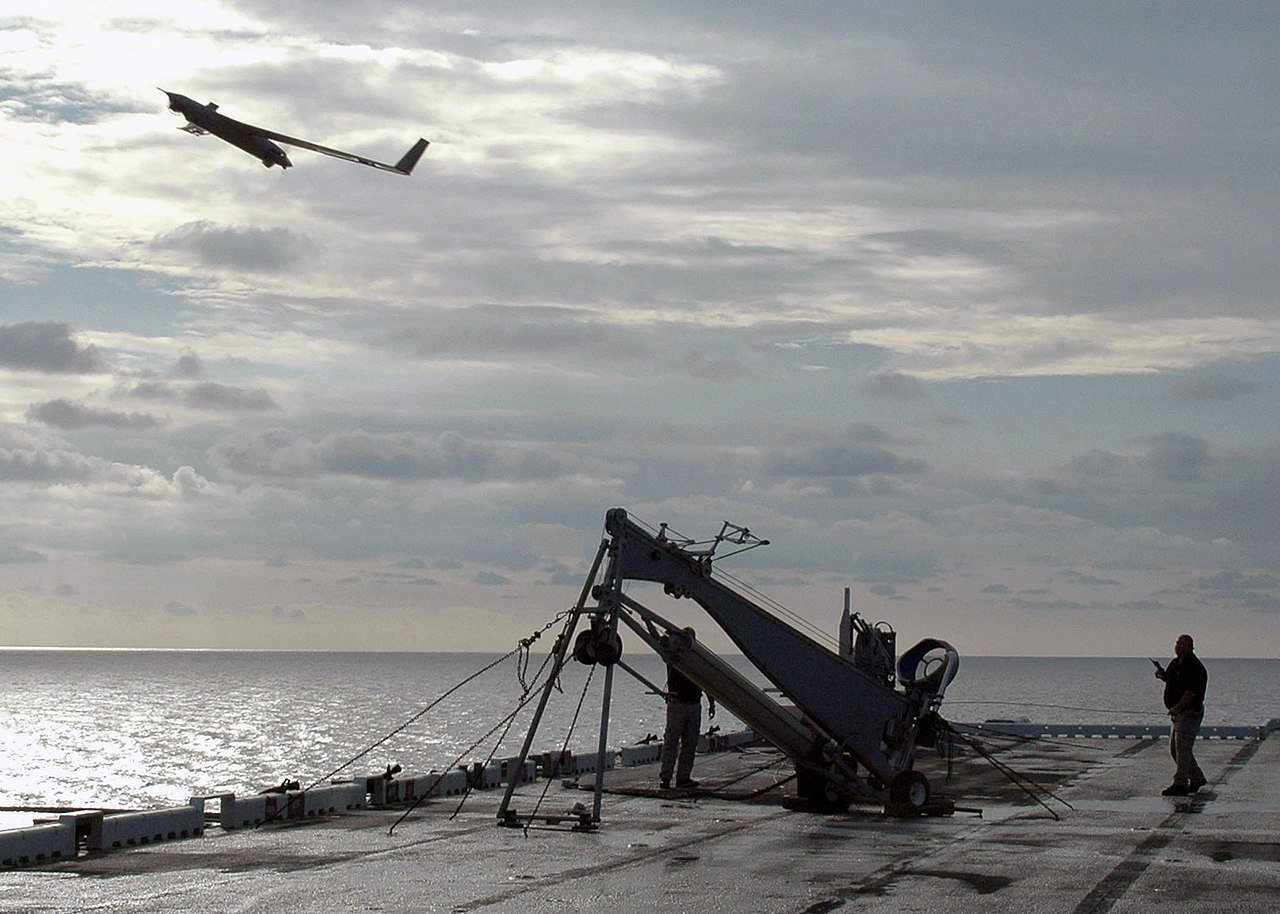
Launch of a ScanEagle UAV, typical of the sorts of threats SEQ-3 might face
But the holy grail of naval lasers is a system capable of defeating more conventional direct threats such as rockets and cruise missiles. While these may not be inherently that much tougher than a boat's motor, they move much faster, giving the laser less time to kill them. Even the relatively slow Harpoon travels at around 240 m/s. While the 30 seconds this speed gives a full-powered HELIOS seems like plenty, in practice the missile doesn't just disappear when it's "killed". It has to be taken out in a way which means that what's left of it isn't a threat to the target. This is a serious problem for existing hard-kill systems, to the point that one of the main drivers of the RIM-116 RAM was the need to take out supersonic anti-ship missiles far enough out that their debris wouldn't hit the firing ship, as it would for missiles shot down by Phalanx. The minimum kill range for a laser is going to vary depending on the exact damage mechanism. The easiest thing to do to a typical anti-ship missile is to burn through the nose and take out the seeker, but this has to be done far enough out that the blinded missile is unlikely to actually hit. A hard kill on a closing missile is considerably more difficult, and could involve burning through enough of the missile to either detonate the warhead or hit something vital to keeping it in the air. In theory, a laser's precision can be used to the defender's advantage, making sure the laser isn't wasted on things which aren't either critical to the missile's flight or on the way there, but this needs good intelligence and modeling, and if it's wrong, it could seriously compromise the effectiveness of the system. And obviously a faster missile makes the whole problem significantly more difficult.
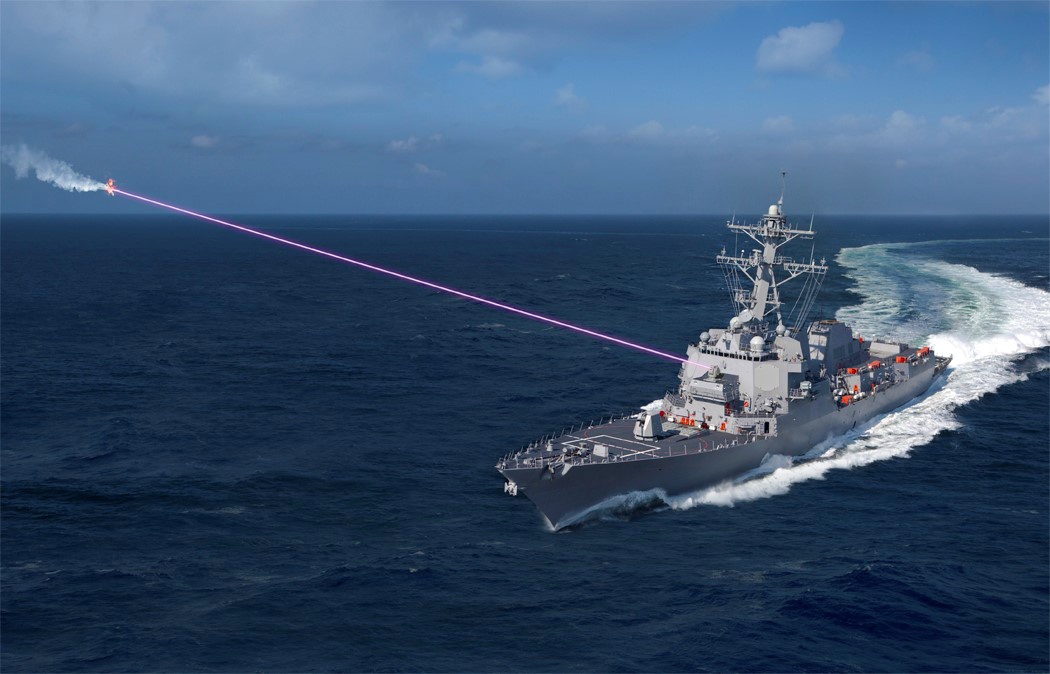
A Burke-mounted HELIOS engages a target9
As a result of all this, current proposals for anti-missile lasers split them into two categories: lasers for handling crossing targets and lasers for handling closing targets. Crossing missiles (which imply that the laser is on an escort, instead of the target ship) are much easier to kill both due to the lack of a minimum range and the ability to target vulnerable areas of the missile from the side. In some cases, this may not even require actual melting/burn through, as ABM laser tests showed that the laser usually caused failure simply by weakening the missile body through heating. The full-power HELIOS might have marginal capability against crossing targets, but most discussion of anti-missile lasers centers around systems with 300 kW or more of power, such as the follow-on HELCAP program. Details on mirror size and power are nonexistant, but it seemed worth analyzing a hypothetical follow-on program. I assumed a 1 m mirror and 500 kW of power, as well as a change in wavelength to 1625 nm, to counter the attenuation that is becoming more of a problem at long range. I also decided to add graphite to my material analysis, as a reasonable approximation of the material on a high-speed missile's nose cone.10 The results show melting ranges of 10.8 km for aluminum, 32.2 km for HDPE, 9.2 km for steel and 12.7 km for graphite, although the drilling rate for graphite at that range is below .05 mm/sec. The full results for our notional laser are in the table below.
Range | Aluminum | HDPE | Steel | Graphite |
---|---|---|---|---|
10 km | 5.89 mm/s | 42.05 mm/s | N/A | 0.12 mm/s |
7.5 km | 15.87 mm/s | 113.28 mm/s | 5.67 mm/s | 0.32 mm/s |
5 km | 55.64 mm/s | 397.26 mm/s | 19.90 mm/s | 1.12 mm/s |
4 km | 104.60 mm/s | 746.89 mm/s | 37.41 mm/s | 2.09 mm/s |
3 km | 224.46 mm/s | 1602.70 mm/s | 80.28 mm/s | 4.49 mm/s |
2 km | 610.10 mm/s | 4356.25 mm/s | 218.20 mm/s | 12.20 mm/s |
1 km | 2935.36 mm/s | 20959 mm/s | 1049.84 mm/s | 58.70 mm/s |
Obviously, the short-range validity of most of these numbers is somewhat questionable, but it does suggest that the generation of lasers currently in the lab will be quite potent anti-missile weapons. While the drilling rate for graphite is quite low,11 it's worth pointing out that graphite ignites at temperatures of around 900 K, well below the sublimation temperature (~4000 K). This is obviously a major problem for any attempt to use it as anti-laser armor in an atmosphere, although the low heat conduction means that the flame may not burn through it very quickly.
But the other takeaway of these numbers is that while lasers are tremendously potent at point-blank range, we're still a long way away from the day when lasers will completely dominate combat. A typical naval laser might be mounted 10m above the waterline, giving a distance to the horizon of about 11.3 km. This is pretty close to what I would call the "effective range" of even the most powerful of the analyzed lasers (not to say it couldn't do anything further out, but it's the point where drilling rates start to get useful) and anything which isn't the surface of the sea would be visible further away. The days when nothing can come within line of sight of a warship without dying are quite a long ways away. There is, of course, one important caveat to this. My model so far has assumed that the beam is propagating close to sea level, with all of the atmospheric effects that implies. This is going to be overly conservative for air targets, and I attempted to compensate for this by creating another tab of the spreadsheet where the effective range for the atmospheric effects is reduced to match the actual amount of air the beam is propagating through.12 To get some idea of what this would mean in practice, I re-ran some of the numbers for the 150 kW HELIOS at several different altitudes:
Range | Altitude | Aluminum | HDPE | Steel |
---|---|---|---|---|
10 km | 100m | N/A | 7.77 mm/s | N/A |
10 km | 500m | N/A | 8.35 mm/s | N/A |
10 km | 3000m | N/A | 12.40 mm/s | N/A |
7.5 km | 100m | N/A | 22.62 mm/s | N/A |
7.5 km | 500m | N/A | 23.99 mm/s | N/A |
7.5 km | 3000m | 4.61 mm/s | 32.93 mm/s | N/A |
5 km | 100m | 12.01 mm/s | 85.73 mm/s | 4.29 mm/s |
5 km | 500m | 12.53 mm/s | 89.48 mm/s | 4.48 mm/s |
5 km | 3000m | 15.71 mm/s | 112.15 mm/s | 5.62 mm/s |
So even attempting to take the thinner atmosphere at high altitudes into account doesn't really change the picture. There's a significant increase in performance, particularly as the targets get to medium altitude, but practical range is unlikely to increase more than a kilometer or two even with that, leaving ranges against airborne targets far short of the horizon.13 But all of this ignores deliberate attempts to frustrate laser use, which we'll look at next time.
1 For those interested, the lasers are combined noncoherently. Essentially, instead of doing a bunch of work to get the waves to line up with each other, they just stuck them together and called it good. ⇑
2 Specifically, the rendering of an SEQ-3/CIWS combination from this report, although I did check it against another actual photo from Ponce, which gave broadly the same result. ⇑
3 I had to use some fudge factors to make my numbers line up with those from the paper. I'm not quite sure why, but I'm going to assume they knew what they were doing, and the basic numbers they gave are correct. ⇑
4 The "will it melt" analysis is quite conservative, as it assumes that the material we are attempting to melt is in an infinitely thick slab, and that we only have a 1-second dwell time. If this is not the case, and particularly if it is instead a thin skin, then melting will occur much earlier. The melting rate analysis, on the other hand, is very optimistic, assuming that the melted material leaves the hole the instant it melts, and carries no extra heat with it. I'd estimate that a more realistic value is half of that shown on the sheet, but I'm going to use this rough calculation throughout. ⇑
5 I'm following How to Build a Laser Death Ray here. Effects of Directed Energy Weapons suggests that a few percent is more common, which seems unlikely given how few things of that absorptivity are found in nature. ⇑
6 Other analytical parameters are wavelength of 1045 nm, Beam Quality 3 and Wind/Slew Velocity 5 m/s. ⇑
7 I am unsure that plastic will actually melt at this range, although it should be pointed out that most materials get weaker when heated, and it's very possible to, say, shoot down a drone by heating the wing to the point that it breaks without actually melting anything. ⇑
8 As this is based on a constant intensity (measured in kW/m2), the melt rates are the same as they are for the same intensity for SEQ-3. ⇑
9 Image courtesy of Lockheed Martin. ⇑
10 Note that in the spreadsheet, the "melting" properties for graphite are actually for sublimation, as Graphite under most conditions tends to go straight from a solid to a gas. ⇑
11 Although I suspect it's much closer to actual values than the drilling rates for the other materials are, as the resulting gas is going to leave immediately. It might well also impose some mechanical stress on the component, which the traditional melting won't. ⇑
12 This is not a great approximation, but it was one that was easy to do and would give more accurate results than completely ignoring the issue. ⇑
Comments
It looks like those LASER weapons have no provision for liquid cooling. Is this correct?
How well would lasers do against something like phenolic resin ablator?
Is that purple beam visible like that, or is that an "artistic enhancement" in the Burke picture? (I'm assuming it's "enhanced for dramatic effect" but I suppose it could be some sort of atmospheric effect?)
I wonder how anti-laser defenses on missiles will evolve as this threat increases. Will they start zig-zagging to throw off the aim if they detect too much heat on their skin, or too much light generally? Will they go for ablation? How will you defend the seeker from getting blinded? (Or do you just let it go blind and rely on a memorized target location and bearing to do final inertial guidance?)
A hundred years from now, when the answers are all public, it'll probably be fascinating.
@ike
No, these are all liquid-cooled. That's standard for naval electronics, so I didn't talk about it.
@Lambert
No idea. From my space warfare days, carbon stuff generally works best, hence my use of graphite, so I'd guess slightly worse than that.
@Ian
Artistic license, I'm pretty sure.
@Alsadius
I'd guess that IR guidance takes a big hit relative to radar. Most radomes are graphite or something of that nature, which is going to work well against lasers and be transparent to radar. I'd guess that ablation is the main defense, but more on that next time.
@Alsadius: Zigzagging costs fuel and makes the missile's targeting solution harder because the seeker's visibility cone will no longer be centered on the target. Plus it stresses the airframe, and may not even work if the laser can predict the zigzags.
Why aren't pulsed lasers used? It would seem creating an explosion on the skin of the target is vastly more destructive than warming it up gradually, even assuming the target doesn't maneuver to distribute the incoming heat (rolling wouldn't even mess with the trajectory).
Pulsing is definitely superior if you can pull it off, but we can't. You'd need far higher peak power than we can get today, and nothing I ran across suggested this is going to change any time soon.
@Ian Argent For a good visual demonstration of laser weapons (and other bits of space warfare), check out Children of a Dead Earth. (Note, a good proportion of the screenshots have things that look like sci-fi lasers. Those are slugs, with the color provided by a tracer compound.) The dev has a blog post on Why does it look like that?
It's hard to imagine an anti-small-boat capability being useful. Won't the laser be completely ineffective against underwater targets?
Anti small-medium drone seems really useful, especially as a means of denying reconnaissance.
Also, have the Israelis been working on something like this for Iron Dome? Could skip the challenges of shipboard integration, co-locate right next to a literal power plant which could be taken off the grid during a rocket attack.
That's why you burn out the engine. The concern here is essentially something like a repeat of the Cole attack, and in that case, it's nice to be able to disable the target without killing them in case you're wrong about what they're up to.
Lasers aren't a great complement to Iron Dome because of limited range. They're pretty good at point defense, not so much at area defense.
I think a good comparison for those ranges is the range of the Phalanx system.
Which wiki tells me is 5 nautical miles = 9 km.
So very comparable numbers.
One of the advantages of the Israelis position, is that they can locate their lasers pretty close to the launch sites in Gaza, and hit the rockets while they're still slow(ish) and full of fuel. That might make lasers more effective for them than in the typical case.
Graphite does have high absorption of the laser beam, ideally you want something that will reflect most of it (and also for that material to be able to take some heat and also conduct some away, Beryllium Oxide fits those requirements pretty well).
Beryllium Oxide? Yeah, that's going to go over very well. At least graphite isn't carcinogenic. And I also wasn't trying to say definitely graphite, so much as using it as a hypothetical laser-resistant material.
Can't crossing targets just roll, thereby denying the laser a consistent place to aim?
And could multiple laser systems (possibly mounted on different vessels) point at the same spot on the same target?
Most missiles have a distinct up and down. Rolling is theoretically possible, but you'd need to build it in from the start. As for coordination, I suppose you could, although I'm not sure how often you'd have multiple ships which have similar enough views for it to work well.
Rolling requires energy - that energy is coming out of the missile's energy budget, and therefore range. Plus, it'll screw with the aerodynamics of the missile body.
What if a 1000 foot warship with a very large electrical generation capacity had laser emitters on multiple sponsons and could put multiple beams on a single target? Would that be a meaningful improvement in melt/kill range, or at least kill rate? Rumor has it this capability is intended for the Ford class …
Multiple beams would spread out the energy compared to a single beam so for engaging a single target you probably don't want to do it but if you're set up to engage multiple targets but only have one you may as well send multiple beams.
Oh, obviously these kinds of countermeasures come out of your energy budget. But getting shot down takes a much bigger percentage of your energy budget. If these become common defensive systems, countermeasures will be found, and the energy budget will be increased as necessary. I just don't know the details of how that'd work in practice.
@jers
It might help a little bit, but my big takeaway from this is that increased power doesn't push the range out as much as you might think. Multiple beams would cut blooming down a little bit relative to a single one, but you're still getting bigger beams at longer range. A 2.5x power boost on HELIOS saw about 25% more range.
Would burning off a missile’s fin work? Are the lasers not precise enough to do that, or is that not a good enough guarantee of stopping it in a timely manner? In theory I’d imagine you could destabilize the missile and get it to veer off course (preferably into the sea) but I’m not sure if that would be something you could achieve consistently, and if it’s not I guess it’s not worth the risk…
Also—has the Navy talked specifically about integrating laser weapons into the future unmanned ships they are eying? Seems like having a low-riding low-signature vessel armed with a laser fore-and-aft (and maybe a RAM?) hovering 10km outside of a Burke to get those crossing shots seems like a good idea and wouldn’t have as much of a problem with enemy jamming or independent action because it would be within LOS as an extension of a manned vessel. Does this seem like a good idea or am I missing something?
If effective range is only weakly dependent on power, and determined more by the thickness of atmosphere than by the geometric distance, that points - I think - toward an airborne system.
Consider a laser system with a range of 6km in sea-level air, mounted on a platform at an altitude of 10km (flight ceiling for an E-2), where the atmospheric density is ~25% of its value at sea level. Against targets at the same altitude, its effective range is 24km. Against sea-skimming targets, it's effective within a circle of radius ~5km centred on the surface point directly below it - and these will, of course, be crossing shots.
This is all a bit marginal: if the laser in the example above had a sea-level range of 5km rather than 6km, the airborne version wouldn't even be able to touch a sea-level target. And my assumption above - defining effective range in terms of atmospheric column density - won't be completely valid.
But if airborne laser platforms do end up being the most practical option, it leads to a rather different picture of naval warfare. CATOBAR carriers become vital to the defense of their escorts: you need catapults to launch big aircraft with high power generation to defend each of your smaller vessels.
@Suvorov
You might be able to do that on a crossing target, but I wouldn't give good odds for a head-on one. As for unmanned ships, I didn't run across any references to it, but that's not a bad idea.
@DampOctopus
That analysis isn't quite the full picture, as you're still going to get diffraction (which I separated out in my math). Also, I'm skeptical of fitting a practical laser into an airplane. It's been done, but only with chemical lasers, which have a lot of issues. The airplane would need not only the laser itself, but also all the power and cooling provided by the host ship, and that seems likely to be too much for the catapults.
How about a kite Laser? Use a kite/tethered balloon to lift the laser, and run the power (or with fiberoptics, perhaps the beam?) up the cable. You wouldn't have the same altitude as an aircraft, but you'd be vastly higher than a ship's deck, or mast.
I had thought of bouncing a ship-mounted laser off a flying mirror to re-collimate the beam, but I like that fibre-optic idea much better. You do need an altitude of a significant fraction of the atmospheric scale height (~7km) to see a benefit from decreased atmospheric density, though.
Fibre attenuation may be a problem: it's ~1dB/km, so after even just 2km, you're down to 63% of your original power. But maybe you can get lower attenuation if you're willing to sacrifice signal fidelity (which is the usual concern for comms fibres).