I've previously discussed the fields of Electronic Signal Measures (ESM) and Electronic Countermeasures (ECM). But now let's turn to the third major branch of electronic warfare, Electronic Counter-Countermeasures (ECCM). ECCM is concerned with defeating or at least mitigating the effectiveness of ECM, through a variety of techniques even more diverse than those used to attack systems in the first place.
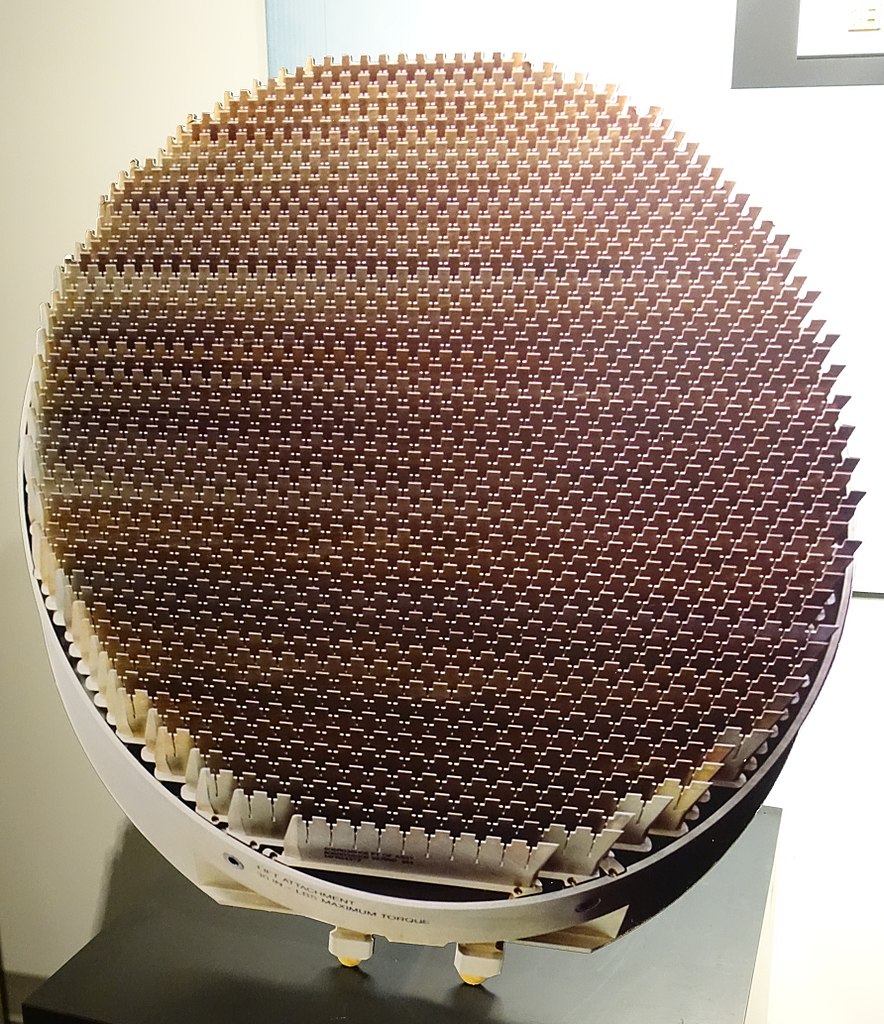
Modern AESA radars like this AN/APG-81 incorporate many ECCM features
The most basic ECCM technique to counter jamming is increasing transmission power. Because radar return strength falls off with the fourth power of range1 while jamming falls off with the square of range, at some point the reflected signal will be stronger than the jammer, and the radar will pick it up. This is a particular problem when airborne platforms attempt to jam surface radars, which have much larger power budgets.
But increased power isn't always available, so designers had to get tricky. The most basic of these techniques is frequency-hopping, where the radar2 changes exactly what frequency it is transmitting on several times every second.3 This renders spot jamming ineffective. Even if the radar does pick a jammed frequency, it will only be jammed for a short time. Barrage jamming can theoretically block a frequency-hopping radar, but only by putting out enough power to essentially spot-jam every single frequency. But what about a frequency-agile spot jammer, you ask? The problem is determining what frequency will be in use next, and changing the jammer to that frequency before the radar switches away. The only real vulnerability is if the pseudorandom code which determines how the radar switches frequencies is compromised.
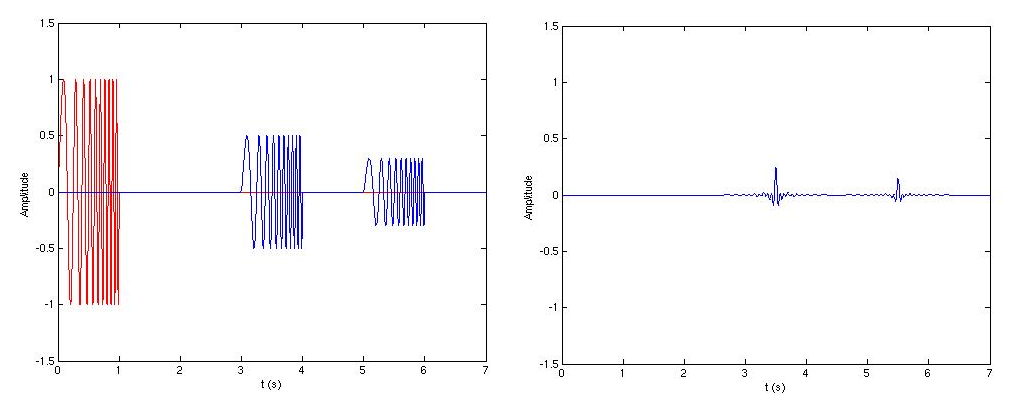
A demonstration of pulse compression. Original signal to the left, compressed signal to the right.
Another important technique is pulse compression, where signal processing is used to increase the radar's resolution and its signal-to-noise ratio.4 Instead of sending pulses at a constant frequency, the radar is "chirped" and transmits a pulse that increases in frequency. Signal processing is then used to compress the pulse by adding delays to lower-frequency returns. Let's say that our radar sends out three discrete pulses, one right after the other, at 101 MHz, 102 MHz and 103 MHz, each lasting .1 microsecond. It then listens for returns on all three bands. When it processes the signal, it delays the 101 MHz signal by .2 microseconds and the 102 MHz signal by .1 microseconds. This will "stack" the returns on all three bands, effectively giving three times the power of the actual transmission, while noise and jamming will be unaffected. This also gives better resolution, as the pulse effectively comes in over .1 microsecond instead of .3.5
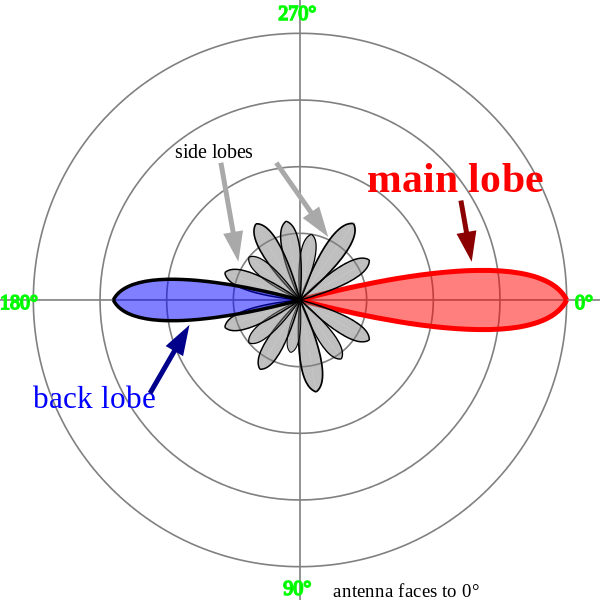
The sidelobe patterns of an antenna
Other techniques besides pulse compression are often used to counter noise jamming. The use of a specific polarization can greatly attenuate jamming done on other polarizations. A radar beam often has sidelobes, areas of relatively high sensitivity in a significantly different direction from the main beam. These provide an opportunity for jamming to sneak in, but an omnidirectional antenna can provide a comparison signal which can pick these up and allow them to be removed. Even better is to design the radar to reduce sidelobes as far as possible. Because a radar assumes that any signals are coming from the direction it is looking, an electronic warfare system in a sidelobe can create false targets. Sidelobe suppression also makes it harder for radar-homing missiles to track a radar, something the crew has a healthy interest in.
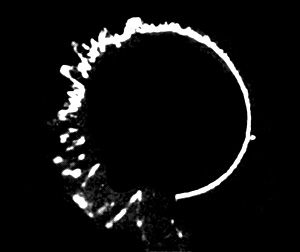
The effect of chaff on a Giant Wurzburg
Many ECCM techniques are also useful in dealing with chaff and various natural returns that might clutter a display. The earliest of these was probably the German Wurzlaus modification to the Wurzburg, developed to counter Window. It used the doppler effect to screen out any echoes moving at less than 13 mph relative to radar. This was a massive improvement over being jammed by Window, but had its own issues. An aircraft flying perpendicular to the line of sight to the radar would not show up, while winds that moved the chaff clouds at more than 13 mph would clutter the screen again. Another system, known as Nurnberg, allowed the operator to listen to the returned signal from a contact. The propellers of a bomber would modulate the signal, producing a rustling sound, while a cloud of chaff would be silent.
More effective ECCM systems were developed later in the war. Instantaneous Automatic Volume Control removed long echoes, from chaff, clutter, or jamming, from the radar screen by reducing the gain of the receiver based on the strength of the previous pulse, delayed by one pulse length so isolated pulses would be unaffected, but long returns would be suppressed. Other techniques more directly suppress echoes significantly longer than the pulse length, which are much more likely to be jamming or unwanted returns, and modulate the radar's overall gain to maintain a so-called Constant False Alarm Rate (CFAR). This means that instead of blanking out the screen, jamming merely pushes down less obvious echoes and makes the radar less sensitive.
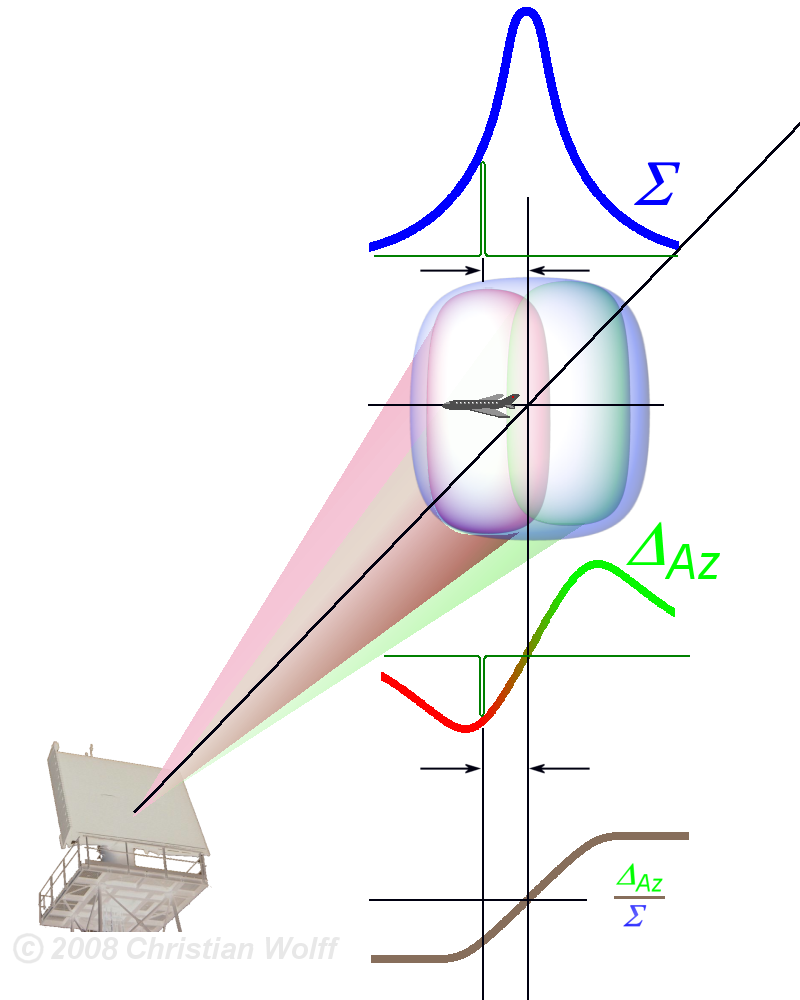
Monopulse radar
Besides techniques designed to counter noise jamming, ECCM has gone a long way to reduce the effectiveness of other ECM techniques. For instance, chaff is ignored by moving target indicator (MTI) radars, which compare the signal received on subsequent passes to filter out stationary targets. More modern pulse-doppler radars can measure velocity directly, making it even easier to distinguish stationary decoys. ECM techniques like angle stealing have also suffered. Angle stealing is particularly effective because the rotation rate of the system can be determined by the receiver, which can then match it at a different phase. An early solution was to only rotate the receiving antenna, denying this information to the target. This was still too vulnerable, and monopulse radars, standard since the 1960s, use signal processing and specialized antennas to compare a single pulse against itself. This makes it much more difficult for a jammer to conduct deception jamming. The signal structure must be replicated fairly precisely, or it will be discarded during processing.6
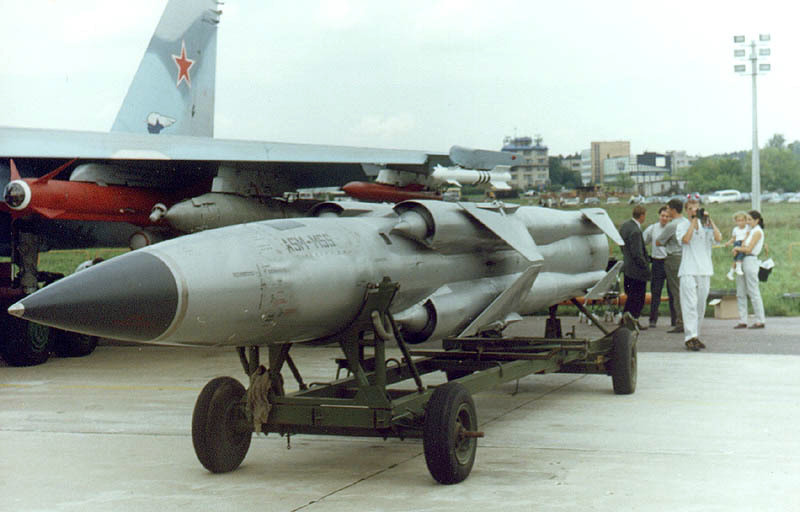
P-270 Moskit anti-ship missile
The advent of digital signal processing has lead to further advances in this kind of target discrimination. Some techniques make use of information about the precise characteristics of tracks, similar to non-cooperative target recognition, to tell the real ones from the fakes. Others use the behavior of the signals to pick out genuine contacts. And computers are increasingly able to integrate data from multiple systems, which makes fooling all of them at the same time very difficult. An early example of this is the method used by many antiship cruise missiles, which have both radar seekers and IR sensors, and are programmed to ignore radar targets which don't also show an IR signature.
Electronic warfare is a massive and complex field, and one I've barely scratched the surface of. From gathering signals to figure out what systems the opposition is using to trying to draw off an incoming anti-ship missile, EW is mostly invisible, but utterly vital to the effectiveness of a modern military.
1 This is essentially because it undergoes two inverse-square attenuations, first on the way out and then on the way back. ⇑
2 Or radio. I haven't discussed much about specifically communications-related EW, but many of these techniques are applicable to that as well. ⇑
3 Interestingly, one of the early inventors of frequency-hopping was actress Hedy Lamarr. ⇑
4 Pulse compression also avoids issues that can crop up at high radar powers, such as the air inside the waveguides breaking down and shorting things out. ⇑
5 A .1 microsecond pulse will be able to distinguish between targets about 30 m apart, as opposed to 90 m for the .3 microsecond pulse, thanks to speed-of-light delays. ⇑
6 Another clever technique was that used by Soviet anti-ship missiles to counter range-gate pull-off. Instead of using every returned pulse, seven out of eight were discarded. As a result, when the receiver did look at another pulse, it was likely that the deception signal would have left the gate, while the target signal would still be in it. ⇑
Comments
One part of the story that isn't mentioned is the way solid state electronics change the way radar works. Solid state has a high ratio of average to peak power, compared to vacuum tubes that have much higher peak powers then average powers. So instead of pulses they use filters to spread out and reconstruct pulses from waveforms that increasingly look like white noise. This makes jamming tricky.
Interesting. I was not aware of that drawback to solid-state systems. Do you think that's part of the reason for things like chirping? Although what you're describing looks more like conventional spread-spectrum stuff. I looked some, and wasn't able to find any direct references to using that in modern radar. But I didn't look that hard, and I remember having heard such things in the past. Maybe it's tied into LPI radar, which I didn't look that closely at.
It is the reason for chirping! Once you have DSP all the waveform tricks get a lot easier to do without lots of expensive hardware.
Could you give some examples of other waveform tricks? I know next to nothing about signal processing, but it's a field that looks like it would be interesting if I could find any references that didn't have several equations per page.